Ice: How the Last Ice Age Shaped Brookline
The last major event to shape the geology of Brookline was the advance of the Laurentide ice sheet in eastern North America. The Laurentide was one of several ice sheets that advanced south in the North American continent starting about 75,000 years ago, an event called the Wisconsin glaciation. From about 34 million years ago, the earth moved into the last of what have been six major "ice ages." (A summary of these periods can be found here.). During the earlier period of this ice age, ice accumulated and expanded on the Antarctic continent. Over the last three million years, the ice mass in the northern polar region has expanded. This period is demarcated by scientists as the Pleistocene period. This is typically what most people (and movie makers) refer to as the ice age. It is the time when humans emerged from Africa and spread across the globe.
Tectonic forces contributed to the triggering of the Cenezoic ice age. By 40 million years ago ("Ma"), plate motion widened the narrow gap between South America and Antarctica, resulting in the opening of the Drake Passage. This allowed for the unrestricted west-to-east flow of water around Antarctica, what scientists call the Antarctic Circumpolar Current, which isolated the southern ocean from the warmer waters of the Pacific, Atlantic, and Indian Oceans. As a result of this change, the Antarctic cooled significantly, leading to the onset of the new ice age with glaciers starting to form on Antarctica.
Around 15 Ma, subduction-related volcanism between Central and South America created the connection between North and South America, preventing water from flowing between the Pacific and Atlantic Oceans. This further restricted the transfer of heat from the tropics to the poles, leading to a rejuvenation of the Antarctic glaciation.
It Wobbles
Over the last three million years, the advance and recession of ice on the earth has been controlled by changes in the earth's rotation--related to irregularities in the shape of the earth--and its orbit around the sun. The existence and duration of these cycles were worked out by the Serbian scientist Milutin Milankovitch in the 19th century. He defined three “wobbles” that happen on a cyclical basis and affect global climate. They are precession—the changing of the axis of spin of the earth, a cycle of 25,771 years; obliquity—the changing of the angle of the earth toward the sun, a cycle of 41,000 years; and eccentricity—the change in the distance of the earth from the sun in its orbit, a cycle that occurs over 100,000 years. NASA has a great explanation of these, so to get more background check out the link here.
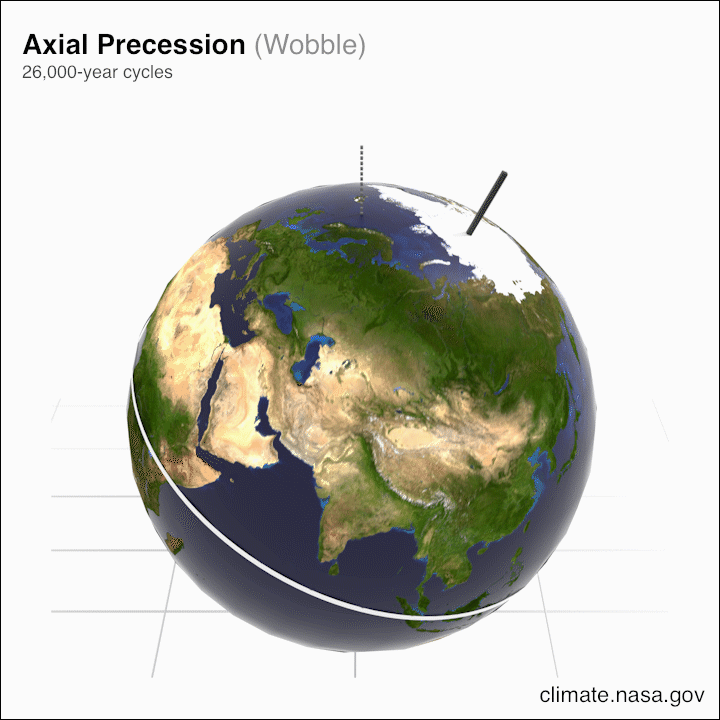
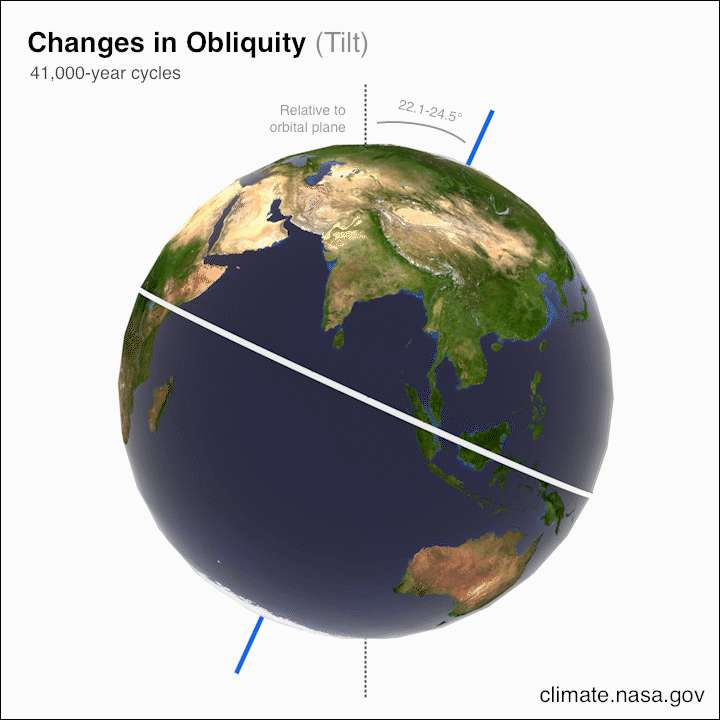
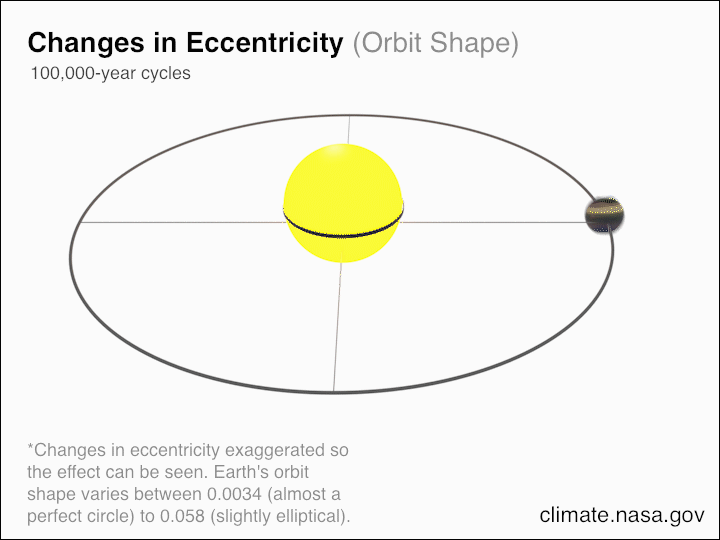
Each of these “wobbles” affects how much solar energy the surface of the earth gets. These factors each contribute to how much ice and snow may be deposited in the colder polar regions. We can think of the combined effect of the Milankovitch cycles as a one-armed bandit in a casino. One of the cycles reaches a maximum at least every 26,000 years or so. Two of the cycles reaches a maximum much less often—like getting two cherries on a slot machine—but a maximum in all three cycles at the same time becomes like hitting the jackpot. This happens only about once every 430,000 years! Looking at changes in temperatures going back over 2 millions years using drilling cores from the Antarctic ice (Figure right), we can see that there is a broad correlation between average temperature and solar irradiation mediated by the Milankovitch cycles.
Right Figure: Correlation between mean temperature and solar irradiation from a series of deep ice cores collected at the Vostok Base in Antarctic. Temperature was extrapolated from changes in ratios of hydrogen and oxygen isotopes in the ice cores, which change proportionately with temperature. Changes in solar irradiation on earth is calculated from the combined results of the three Milankovitch cycles. Image from: http://www.climatedata.info/forcing/milankovitch-cycles/.
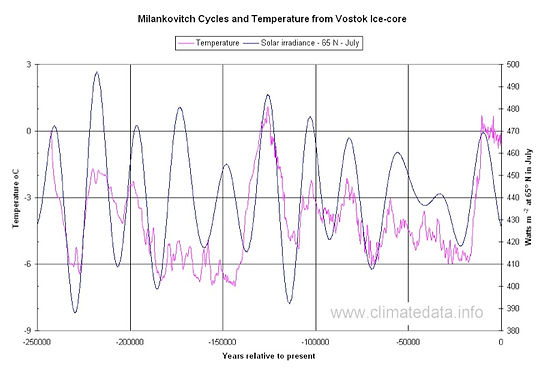
A Critical Ice Mass
The Milankovitch cycles individually can contribute to warming or cooling of the planet. During so-called maximums in a cycle, the cooling of the planet may be favored, with the resulting effect that winter season growth in regional ice cap or glaciers is not reversed during the following summer, leading to an accumulation of ice. The more surface area that is covered by ice, the more solar energy is reflected back into space, a feedback process that can accelerate ice formation.
By the Quaternary period of the Pleistocene—a geologic era of time between 2.6 million and about 12,000 years ago—large ice sheets formed in and around the two continents located near the north pole: North America and Eurasia. In keeping with the Milankovitch cycles, these ice sheets advanced or retreated on a continental basis.
There were four main ice advances during the Quaternary: The Nebraskan, The Kansan, The Illinoisan, and the Wisconsin, which started around 75,000 years ago and lasted until about 11,000 years ago. A number of regional ice sheets were generated during this time, dominated by the Laurentide ice sheet. At maximum, the North American ice sheets grew to a depth of more than two miles and covered about 5.7 million square miles.
Ice is different than many solids. Under pressure, it exists in a plastic state, and can bend and flow. The incredible weight of two miles of ice squeezed ice out at the edges of the sheet, pushing southward along a broad swath of North America. At its greatest extent, the North American ice sheet reached near the US-Canadian border in the West. In the Midwest several huge lobes of ice pushed south as far as central Iowa, Illinois, Indiana and Ohio. On the east coast the Laurentide ice sheet pushed out beyond the current borders of New England into the ocean, and as far south as northern Pennsylvania.
Image: Maximum coverage of the North American ice sheets, around 15 thousand years ago. Image from: https://opentextbc.ca/geology/chapter/16-1-glacial-periods-in-earths-history/
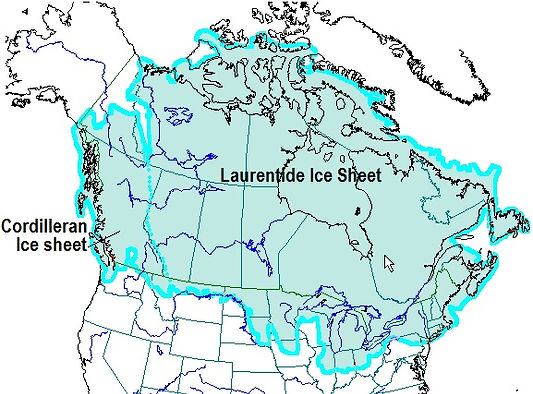
During this glaciation, so much water was locked up in ice that the sea level dropped over 300 feet. The weight of the ice sheet was so massive, it pushed the underlying continental rock down into the mantle like one might push a cork down into the water. Under the thickest ice in Canada, the crust was pushed down almost 3,300 feet, flooding parts of northern Canada under the northern Atlantic Ocean. After the ice melted back, the earth slowly rebounded from this weight. The ice sheet also acted as a continental bulldozer, scraping off billions of tons of sediment, scouring out huge gashes in the earth (the Great Lakes), and rounding off the underlying rock. It wasn’t just the weight of the ice that had this effect, it was all the sand and rock that was picked up into the ice along the way. Much of this was retained at the bottom of the ice mass and acted as a continental sandpaper that scoured and smoothed off the earth’s surface under the weight of the ice. We can see the results of this scouring effect throughout the rocky landscape of New England.
The furthest advances of the ice in Massachusetts occurred by around 20,000 years ago, with the edges of the ice sheet somewhat south of Cape Cod (Cape Cod itself is the terminal moraine of one lobe of the ice sheet). After that, ice began to retreat as the climate began to warm. This retreat was gradual and marked by a series of advances and retreats of the ice. By about 16,000 years ago, the Boston area was ice free. Later, between about 12,200 and 11,600 years, the ice made another advance. After this, the ice sheet fully melted back, leaving a landscape that did not look anything like it did before the start of the advance. We can still see the signs of the last ice age in the features of Brookline.
Under the Ice
The features that are left in the surface geography of the Brookline area are a result of what happened under the ice surface, both during the advance of the ice sheet and its retreat. To understand this, we should consider several factors.
First, ice flows in advance but not in retreat. The flow of ice from the north was an effect of gravity, with ice flowing out from the high elevation areas in the north. While some of the growth in the ice sheet was a result of snow and ice formation, the bulk of the destructive effect of the ice sheet was a one-way event. Lobes of ice pushed southward until the gravitational force was offset by the friction presented by the ground and the warmer temperatures of the more southern latitudes. Once the ice sheet reached a point of stasis, the retreat was due to melting, not backward movement. This means that all the sediment and rock picked up by the ice sheet was a “one way” delivery. Once the ice sheet stopped moving, retreat was in fact melting, and the sediment and rock caught up within the ice began to drop out on the ground. Some of the local features of Brookline are the result of sediment and rock dropping out of the ice during the melting/retreat of the ice sheet.
Second, ice sheets are complex inside. We often think of the ice age as being caused by a giant block of ice pushing everything out of its path as it made its way south. But the reality is much more complex. Ice sheets and glaciers, which are in essence smaller ice sheets, are not completely solid. They have large gaps formed by cracks and crevices which appear when ice fractures instead of flows. They also develop internal lakes—pools of unfrozen water—and rivers, where ice melt results in internal flows within the ice. These internal rivers can redistribute sediment inside a glacier, just like a river redistributes sediment from the land. As a result of the irregularities within the glacier, some parts may be loaded with sediment, and other parts not. Some parts might be full of dirt and sand, while other parts might be loaded with larger size rocks, and even massive boulders. The internal structure of the glacier matters when it reaches its maximum coverage and begins to recede. In areas were internal rivers breach the end of the ice sheet, sediment can be carried out onto the earth. In other areas, large solid blocks of ice may be surrounded by more porous ice. As the ice sheet melts back, these blocks of solid ice are left behind, leaving an impressions in the ground as sediment dropping out of the ice sheet, or flowing out of the ice, are deposited around it.
At the edge of the ice sheet (Brookline was inarguably at such an edge), glacial retreat was not a steady process. Rather, between about 17,000 and 9,000 years, retreat was a cyclical process of advance and retreat. This means that some areas experienced initial sediment deposition, which were then reworked and redistributed by subsequent glacial advance. That can make the interpretation of the local geology somewhat more complex.
Ice Sheet "Fossils"
Fossils are the preserved remains of living organisms in the rock. Sometimes these fossils are of the structure of the animal or its skeleton, and sometimes it is the signs of its activity—footprints, burrows, even its excrement. In an analogous manner, the modern landscape of Brookline and surrounding Boston Basin bears the “fossil” imprints of the Laurentide ice sheet. This section describes specific glacial features of ice sheet advance and retreat that can now be found in the surrounding area.
Rock erosion/smoothing: Ice sheet advance typically smooths the underlying rock. Depending on differences in the outcrop height and composition, the result is an undulating, rounded surface, with smoothing tending to be more gradual on the side of the rock in the direction of origin (the so-called stoss side) and sharper on the direction of flow (the so-called lee side). On the lee side, the force of the ice against the rock face can result in rock being pulled out of the deposit ("plucking"), resulting in a sharper, more ragged edge. This resulting, distinctive shape is called a roche moutonee or sheepback.
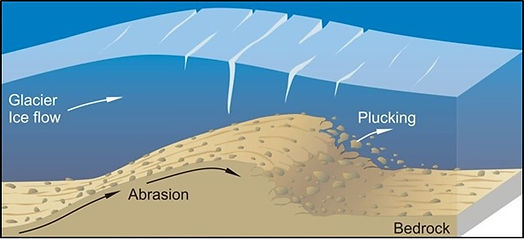
Glacial scouring: Ice sheets carry large amounts of sediment in the bottom layer that has been picked up by the movement of the glacier. These sediments include mud, sand, pebbles, cobbles, and even boulders. The moving ice mass can grind these sediments against one another, further breaking down and smoothing them, but the ice mass also acts as a giant sandpaper, leaving scratches on the rocks over which it passes. These can be relatively fine or in the shape of large gashes if made by heavy boulders with high hardness, like granite. The scratches provide directionality for the flow of the ice. At a regional level, these can provide an overall direction of flow for the ice mass. At a more local level, however, these flow directions can be more variable. This is especially the case when the ice retreats and then advances again, which may result in a different flow direction compared to the first advance.
Drumlins: When the advancing ice sheet reaches a stasis point, and begins to melt back, sediment within the ice sheet does not travel back with it, but is left on the ground over which the ice sheet traveled. Billions of tons of sediment were transported south during the Wisconsin glaciation, sometimes producing rich soils south of the glacier. In New England, one of the legacies of glaciation was a plethora of rocks and boulders, which over the years ended up in the area’s famous stone fences. In some areas of the glacier, large deposits of sediment are concentrated in one area, and deposited as mounds of sediment called drumlins. These hills of sediment tended to lens shaped, preserving the directionality of glacial flow as the longer axis of the hill, and also tending to be more gradual in elevation on the stoss side and steeper on the lee side. The Boston area has more than 200 drumlins, with many of them existing in the rarer form of drumlin “swarms” (including a swarm in Brookline!). A large percentage of the Boston Harbor inner islands are drumlins, forming the only drumlin swarm in the United States that intersects a coastline. Some of the harbor drumlins were first formed in the Illinoisan glaciation, and then added to by the Wisconsin glaciation.

Skehan, SJ. Puddingstone, Drumlins, and Ancient Volcanoes: A Geological Field Guide Along Historic Trails of Greater Boston. Second edition. WesStone Press, 1979, p. xii.
Kettles: Kettles are formed by big chunks of ice that are left as the ice sheet retreats. They are the more solid ice within what is a Swiss cheese-like lattice of cracks, holes, internal rivers and lakes, and piles of debris. These chunks land on the ground in the retreating glacier and are surrounded by the sediment melting out of the glacier or being carried out by streams and rivers. This sediment surrounds the ice, preserving it from quickly melting. Over years, however, the ice melts away, leaving a hole called a kettle. Often these extend below the water table and are filled partially with water, forming a kettle pond. There are several such ponds in the Boston area, including Lost Pond in Brookline, Hammond Pond in Newton, and Jamaica Pond in Boston. Jamaica Pond is paired with a set of nearby drumlin deposits. These ponds are highly variable in size and shape, depending on the size and shape of the leftover ice. Jamaica Pond is over a half mile across and has a variable bottom that can reach almost 60 feet deep at one point, whereas Hammond Pond is only about 1200 feet across at the widest with a relatively uniform depth of only about 4 feet.

Figure from: https://www.geocaching.com/geocache/GC5BGB5_a-different-kettle-of-fish?guid=c49a6b2b-3019-4fc7-97ea-90a94ece6c17.
Glacial erratics: These are large boulders that are carried from one area and left in another by the glacier. The geology of the erratic may be quite different than that of the area where it is deposited. There are a number of famous glacial erratics in New England, including Plymouth Rock, but very few in the immediate area around Brookline. The rock featured on the home page of this site, while small, is a glacial erratic, as it represents Brookline Member of the Roxbury Conglomerate carried from the north and deposited on top of volcanic basalt.
These are ice age features common to the Brookline area. However, there are numerous other glacial features that have shaped the geography of Massachusetts and the east coast, including he formation of large glacial lakes, extensive outwash plain deposits, and terminal moraines like the one that formed Cape Cod. For more on these types of glacial features, click here.